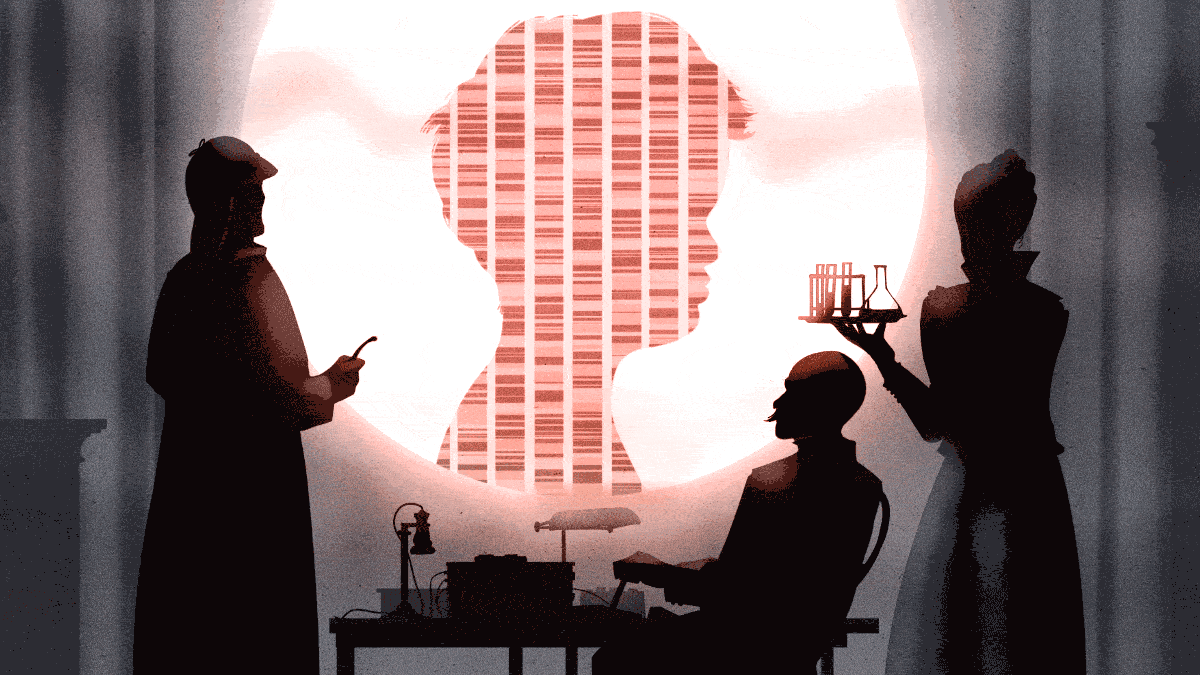
Credit: Daniel Stolle
Emma Broadbent had just turned one when her parents were told that, despite months of tests, physicians still had no explanation for her condition. Emma’s brain was not developing myelin, the fatty sheath that protects nerve axons, and she was not meeting developmental milestones. A host of other issues made eating and sleeping constant challenges. Twice in 2016, the family travelled thousands of miles from their home in Texas to the Children’s Hospital of Philadelphia in Pennsylvania. There, researchers scoured all the protein-coding genes in Emma’s DNA — known as the exome — and came up with nothing. Emma’s parents were told that she had a rare disease but that its exact identity must be hidden somewhere else in her genome.
Part of Nature Outlook: Medical diagnostics
Although individually rare, diseases such as Emma’s are collectively common, affecting about 5% of people worldwide. About 80% of rare diseases are genetic. In their search for disease-causing genetic variants, clinicians regularly refer people for sequencing of pre-determined sets of genes called panels — and more recently, entire exomes. But, although exome sequencing has shortened the diagnostic odyssey for some individuals and families, it still leaves as many as 75% of cases unsolved.
That should be no surprise. The exome, after all, comprises only 1.5% of the human genome. The other 98.5% might not code for proteins, but it does contain instructions for how much of each gene should be turned into messenger RNA, and then into protein. Variations in these parts of the genome can cause disease just as easily as those in protein-coding regions. Examining only the exome is like searching for a single typo in an entire library, but limiting your search to books in the classics section.
Enter whole-genome sequencing, also called genome sequencing, which can capture abnormalities in the exome and non-coding portions of the genome alike. “One test has the ability to basically replace most of the other tests that have been developed and offered throughout our history of genomic diagnoses,” says Brendan Lee, a clinical geneticist and researcher at Baylor College of Medicine in Houston, Texas (see ‘A comparison of common genetic tests’).
This technique has been an option for US clinicians since 2018. To begin with, they rarely called on it, because it was more costly and complex than exome sequencing or gene panels. “It was sort of your last-ditch effort,” says Monica Wojcik, a neonatologist and clinical geneticist at Boston Children’s Hospital in Massachusetts. But with the test now costing less than US$1,000, and new tools to mine the genome becoming available, many think that whole-genome sequencing should be offered much earlier in the diagnostic process. “Now we’re saying, let’s just start with the [whole] genome,” Wojcik says.
In the past five years, a surge of studies has demonstrated how whole-genome sequencing can provide answers to individuals and for entire populations. Its use in the United States is still sporadic, with clinicians struggling to get the test reimbursed by private and government health insurers. In the United Kingdom and some European countries, by contrast, whole-genome sequencing is now widely available to individuals with suspected genetic diseases and cancers through national health services. But even so, the analysis can be limited, leaving large swathes of non-protein-coding regions unexamined. “The whole genome sequence needs to be interrogated to have the best chance of getting a genetic diagnosis,” says Jenny Taylor, who studies human genomics at the University of Oxford, UK.
While some researchers work to promote whole-genome sequencing as a first-tier diagnostic tool for rare diseases, Taylor and others are developing bioinformatic techniques to help to make the most of the data that the test generates. Still others aim to extend the test’s availability to under-served populations, and in doing so, improve the test’s usefulness for everyone.
A diagnostic dilemma
Emma Broadbent’s parents knew almost immediately that she was different. She was ‘floppy’, and facial differences such as her widely-set eyes and low-set ears stood in clear contrast to the appearance of her older sister. “She was super cute,” says Brian Broadbent, Emma’s father. But he knew in a few weeks that caring for her was going to be tough. She spent time in the neonatal intensive care unit (NICU) for lung problems. After going home, she still struggled to eat, pass stool and even breathe. “Sometimes she would hold her breath and turn blue,” her father recalls.
When Emma was five months old, the Broadbents received a call late on a Friday night. As they were expecting, it was not good news. Emma’s physician confirmed that myelin was not developing in Emma’s body and she probably had a type of condition called leukodystrophy. The next morning, Broadbent says, “my fight-or-flight kicked in and I got on clinicaltrials.gov”. He found a trial being run by neurologist and leukodystrophy specialist Adeline Vanderver at the Children’s Hospital of Philadelphia. Vanderver was testing whether whole-genome sequencing could help people with suspected leukodystrophies to receive a genetic diagnosis faster.
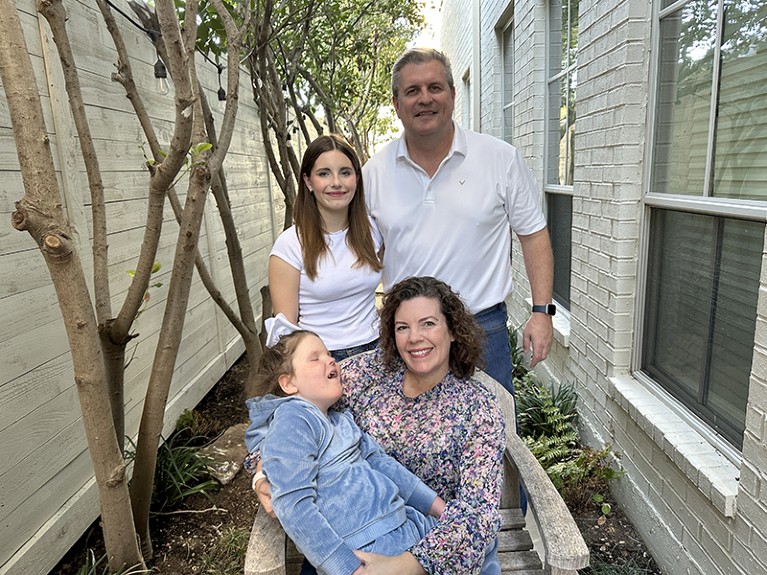
Emma Broadbent (front) with her parents and sister.Credit: Courtesy of the Broadbent family
While Emma was waiting to start the trial, her neurologist in Dallas, Texas, referred her for exome sequencing, which revealed no disease-causing variants. The Broadbents were disappointed to learn that having had this sequencing, Emma was disqualified from the whole-genome sequencing trial. Instead, Vanderver offered to do a full diagnostic examination short of whole-genome sequencing. “We went through everything: metabolic testing, X-rays, she reanalysed our exome data. And at the end of that, we had absolutely nothing,” Broadbent says.
Not ready to give up, he contacted two organizations that offer free genomic sequencing and analysis: the Rare Genomes Project at the Broad Institute of MIT and Harvard in Cambridge, Massachusetts, and the Undiagnosed Diseases Network (UDN), which is funded by the US National Institutes of Health (NIH), and undertakes detailed clinical assessments by teams of specialists that are brought together for each case.
The UDN team found a variant in the code adjacent to a gene called CHD2. Deficiency of CHD2 is known to cause several neurological disorders, but this was not Emma’s problem. Her cells contained extra CHD2 mRNA — the gene was not deficient, but rather overactive. The Broadbents joined a support group on the social- media site Facebook for CHD2-related diseases, and found that Emma’s symptoms didn’t match those reported by other families. This discovery prompted researchers at the Rare Genomes Project to take a closer look at the area of Emma’s genome surrounding CHD2.
Vijay Ganesh, a neurologist at Brigham and Women’s hospital in Boston, who worked on Emma’s data as part of his postdoctoral fellowship, found a deletion further upstream of CHD2, in a gene called CHASERR that encodes a long non-coding RNA (see ‘The whole-genome advantage’). As it turns out, CHASERR modulates CHD2, so its deletion led to Emma’s cells making too much CHD2 protein. The research team posted its findings to Matchmaker Exchange, a data-sharing site that helps to connect the profiles of people with the same disorder or genetic variant. Within two years, the researchers had found two more cases like Emma’s, allowing them to describe what turned out to be one of the earliest examples of a disease caused by a long non-coding RNA variant1.
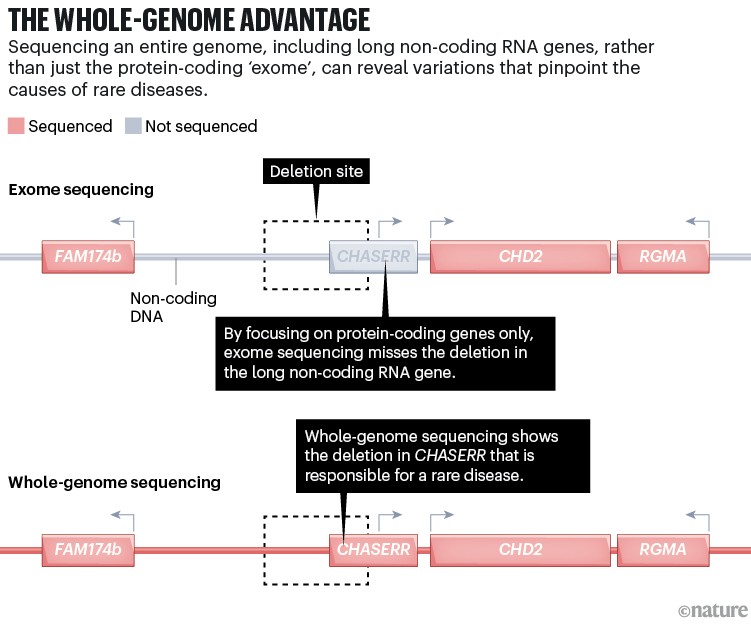
Credit: Alisdair Macdonald
“It was all worth it just to know there were other families out there and that we weren’t alone,” says Broadbent, who stays in touch with the other families through a WhatsApp group. Knowing the cause of Emma’s disorder, which is called Lennox–Gastaut Syndrome, has also lifted some of the uncertainty that was hanging over the Broadbents. “We don’t know what the future holds, but we’ve at least eliminated some unknowns,” he says.
All in the analysis
In his time at Baylor running a clinical-genetics laboratory, which sequences samples for the UDN, Lee has lost count of the number of cases for which whole-genome sequencing provided answers when exome sequencing could not. “It happens all the time,” he says. In 2021, the UDN reported that whole-genome sequencing had diagnosed 41 of 67 patients who had come to the programme after exome sequencing failed to find a causative variant2.
Vanderver says that whole-genome sequencing has completely changed the leukodystrophy field, allowing her to diagnose around 90% of cases, compared with 40% two decades ago. “And there are probably dozens, if not hundreds, more diseases hidden in those non-coding sequences,” she says.
Last year, the US clinical-testing company Revvity reported a retrospective analysis on genomic-sequencing outcomes for 2,100 individuals. The company uncovered pathogenic or potentially pathogenic variants in 28% of people; around 9% of whom would have been missed without whole-genome sequencing3.
Detecting hidden brain injuries
Wojcik led a similar study applying genome analysis to 744 unsolved cases. She and her team identified definite or probable genetic causes of disease for 218 (ref. 4). Of those, around 8% could not have been solved without whole-genome sequencing.
Heidi Rehm, a geneticist and researcher at Massachusetts General Hospital in Boston, who co-led Wojcik’s study, thinks that these studies underestimate how many more cases whole-genome sequencing could solve if given the chance. “I do believe the added value of a genome is far greater than 8%, but we can’t yet interpret most of the variation in the non-coding regions,” Rehm says.
A person’s genome could contain five million variants, most of which are harmless. Using databases and reference genomes, scientists weed out common variants and home in on those with the greatest potential to cause disease. The end result varies depending on where a lab focuses its analysis. “Genome sequencing carries with it this impression that because you’re capturing all that DNA, that you’re looking at all of it equally, and that’s just not the case,” Ganesh says. In clinical analyses, for example, long non-coding RNAs, such as CHASERR are usually ignored, because most of these molecules contain lots of harmless variation; only a fraction are crucial for cell functions.
In a 2023 study, Taylor co-led an international team that delved into genomic data from 122 unsolved rare-disease cases5. By combining a variety of algorithms, the researchers were able to solve 43 of the cases. Many of the disease-causing variants they found fell within introns, which are chunks of non-coding DNA interspersed throughout a gene along with the coding portion, or exons. Normally, when DNA is transcribed into mRNA, the introns are removed. But a change to the intron code can alter this ‘splicing’ process and thereby disrupt the production of mRNA and proteins. Exome sequencing includes splice sites that lie within a few dozen base pairs of the exon, but disease-causing variants can lurk much deeper in the intron, as was the case for 15 of the variants that Wojcik’s team uncovered.
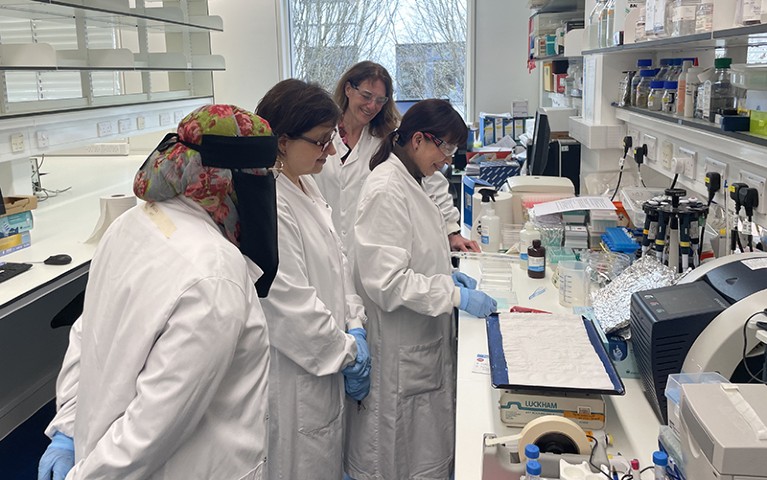
Geneticist Jenny Taylor (centre back) and her team.
Taylor says that this kind of deep analysis is not yet practical on a large scale. In the United Kingdom, although people with rare diseases routinely undergo whole-genome sequencing, the initial analysis is limited to genes that are known to be associated with particular diseases. Through a government-owned organization called Genomics England, individuals can release anonymized data to researchers who can then make deeper queries and get in touch with a person’s physician if they find something new.
In one remarkable example of this process, two research teams independently examined data from the UK 100,000 Genomes Project and identified variants in a small nuclear RNA-encoding gene called RNU4-2 as a major cause of rare neurodevelopmental disorders6,7. One team identified 73 cases and another 115 by studying overlapping data sets compiled from several countries. Both teams estimated that variants in RNU4–2 cause 0.4% of neurodevelopmental disorders. “That diagnosis was there in everybody’s genomes for who knows how long,” Ganesh says. Thanks to these studies, hundreds of people now have a name for their condition — ReNU syndrome — and are building a support network.
Genome first
A concrete diagnosis that involves a new gene variant often requires extra steps such as RNA sequencing, or growing cells and measuring protein levels — as was done for Emma. But, Ganesh says, “our work on the Rare Genomes Project shows that many of these diagnoses just needed a genome. Why should these patients be on such a diagnostic odyssey when you have a viable test that could be obtained and interpreted in quite straightforward ways to obtain slam-dunk causal variants?”
People with rare diseases spend, on average, five years searching for a diagnosis. Many researchers and clinicians think that a ‘genome first’ approach could both shorten that time and save money by cutting out serial testing. Last year, a consortium of US institutions and labs called the Medical Genome Initiative called for policies to support equitable access and universal insurance coverage for whole-genome sequencing as a first-tier diagnostic test for rare diseases. Rehm, who serves on the initiative’s steering committee, says that gene panels are appropriate when clinical assessment clearly points to a likely diagnosis, but “anything where the clinical diagnosis is evolving and potentially ambiguous really should be done on a genome”.
Whole-genome sequencing could also reduce the need for an expert when choosing which genetic tests to refer an individual for, Lee says. His group has received a grant from the US National Human Genome Research Institute to extend whole-genome and RNA sequencing to NICUs in rural Texas, where people have little or no access to genetic testing or analysis. The project will use telehealth to provide genetic counselling and guide local providers through the diagnostic process.
On a global scale, the non-profit organization Genetic Alliance in Damascus, Maryland, is making whole-genome sequencing available in low- and middle-income countries through a programme called iHope. The goal is to cover the costs of diagnosing children with rare diseases, but the project has extra benefits as well — in particular, helping to uncover disease-related variants through sites such as Matchmaker Exchange, and improving the diversity and richness of global genomic databases, when families opt to release their data for research.
Broadbent, who is now co-president of the Coalition to Cure CHD2, says that part of the drive to keep up the search for Emma’s diagnosis was knowing that not all families have the resources to do the same. “I felt a responsibility to both my daughter and other families to push it as far as I could,” he says.
Broadbent is especially proud of the fact that Emma’s diagnosis has opened therapeutic avenues for treating CHD2 deficiency. “CHASERR is not just a genetic discovery for our family,” he says. And although there is little chance that the discovery of CHASERR will lead to a cure for Emma, who, at eight years old, is unable to walk or talk, he says “the probability of curing something if you don’t know what it is is zero”.