Among the most prolific young researchers for cancer-related output in the Nature Index, these rising stars are bringing fresh perspectives to challenges in drug development. From spiky nanoparticles that stick to cells, to drugs that react when exposed to X-rays to boost the effects of radiotherapy, these approaches could become powerful new weapons in the arsenal used to fight cancer.
NAMHEE KIM: Model maker
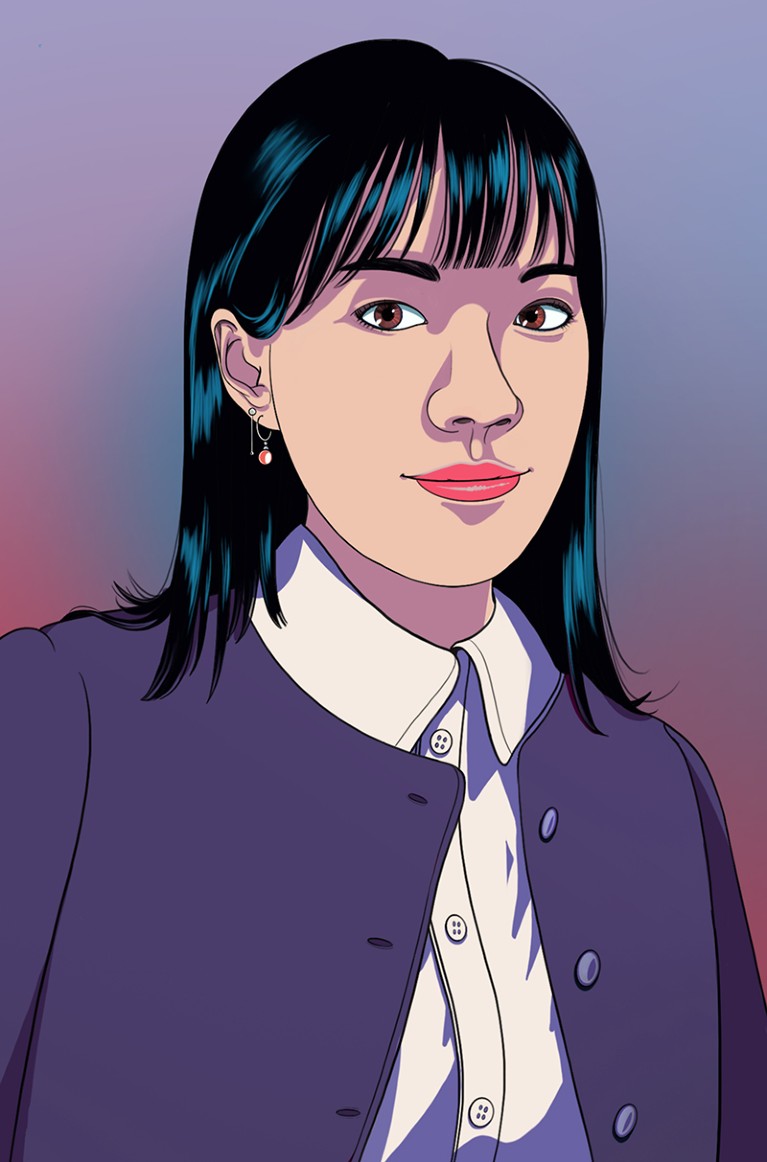
Illustration: Janelle Barone
Namhee Kim doesn’t want to kill tumour cells — she wants to reprogram them to a normal, benign state. If this process, known as cancer reversion, can be triggered effectively, it could reduce the need for debilitating treatments such as chemotherapy and radiotherapy.
As a computational biologist at biotechnology start-up Biorevert in Daejeon, South Korea, Kim is hunting for molecular switches that can induce cancer reversion. Key to this work is controlling a process known as epithelial-to-mesenchymal transition (EMT), which occurs during events such as embryonic development, wound healing and cancer progression. Cells switch from an epithelial state, in which they are tightly packed and stationary, to being more mobile in a mesenchymal state. In cancer, this helps malignant cells to spread more easily throughout the body.
Kim is developing algorithms from large data sets to simulate how different components within a biological system might interact and function as a whole, to identify the molecular switches. “It’s a very powerful way to research cancer and is very fascinating to me,” she says.
Nature Index 2025 Cancer
The work builds on previous research by Kim and colleagues at the Korea Advanced Institute of Science and Technology (KAIST) in Daejeon. They developed a mathematical model for EMT and ran it through a computer simulation to see how it would play out in lung cancer. The research revealed three molecular targets that could potentially switch lung-cancer cells back to a benign state1. They then followed this up with cell experiments to confirm their findings.
In a separate study2, published last year, Kim and the team at KAIST investigated ways to manipulate ‘master regulators’ — proteins that control the expression of large groups of genes and so can direct certain biological processes and pathways within cells. In stem cells, for example, master regulators can dictate whether a cell will become a muscle cell, a neuron or another type of cell by binding to specific DNA sequences and switching certain genes on or off.
Kim and her colleagues tested their method by using master regulators to change mouse cells into different types of cells. Then, they used a cancer network model to show how certain therapeutics could be used to manipulate master regulators in cancer cells to convert them from a malignant to a non-metastasizing state. Once the master regulator is identified, one or more drugs — or combinations of drugs — can be selected for potential use in cancer therapies, says Kim, who joined Biorevert last year to continue to her work.
Kim says she enjoys working in industry because it allows her to “close the gap” between hypothesis and reality. She expects artificial intelligence (AI) to transform her field, aiding in tasks such as data analysis and systems modelling, but says the results can often be difficult to interpret. “We researchers still need to integrate our systems biology background and analytical skills with AI to improve its interpretations of complex cancer systems.” — Sandy Ong
ZIWAN XU: Cancer sensitizers
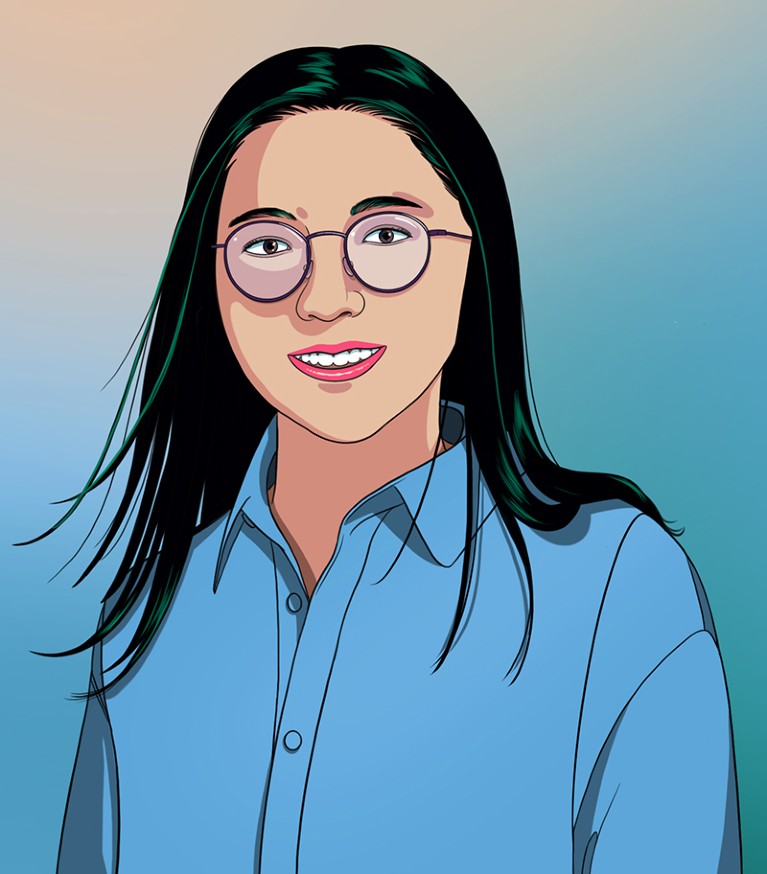
Illustration: Janelle Barone
Ziwan Xu made her mark by coming up with new materials for radiosensitizers — compounds used alongside radiotherapy to enhance its effectiveness against cancer. Radiosensitizers can be made from many different materials, depending on the specific function that researchers want them to perform. Cisplatin, for example, used in the treatment of several types of cancers including cervical, lung and ovarian, is made from platinum. NBTXR3, a radiosensitizer composed of hafnium oxide, helps to treat soft tissue sarcomas — cancers that originate in soft tissues such as muscles, tendons, fat, nerves and blood vessels.
As a PhD student at the University of Chicago in Illinois, Xu developed a radiosensitizer that incorporates thorium, a naturally occurring heavy metal. When thorium is exposed to certain types of radiation, such as that used in radiation therapy, it produces additional radiation and unstable molecules called reactive oxygen species (ROS), both of which can damage cancer cells. The radiosensitizer also incorporates photosensitizing molecules called ligands, which produce extra ROS when exposed to radiation. Together these elements boost the damage dealt to cancer cells by radiation therapy without requiring a higher dose.
To design the radiosensitizer compound, Xu used a technique known as Monte Carlo simulation, which is used to model the probability of various outcomes. “The Monte Carlo simulation work was actually a project I initiated during the pandemic, when we had no access to the lab,” says Xu. She and her colleagues were later able to test the effectiveness of the design in experiments. They injected the thorium-based material into mice undergoing radiotherapy and found that it helped to suppress the growth of colon cancer and pancreatic tumours, compared with a control group that was also exposed to radiation3.
In 2023, Xu took on a role at the pharmaceutical company Merck, based in Rahway, New Jersey, to study another challenge in cancer drug-making: designing injectables that can remain active in the body for long periods. These drugs need to be made of crystals that are large enough that they dissolve slowly in the body, but not so big that they clog the needle when administered, she says. A patient’s immune response to the injectable can change over time, too, which can lead to complications.
Xu says she feels lucky to get advice from experts in many fields at Merck. “Someone can give me suggestions on the analytical methods I use, and someone can brainstorm with me on different instrumentations and different simulation methods,” she says. “You can always get support. Working at the universities, I had collaborators, but it was mostly limited to our lab.”
Having moved to the United States from China in 2017 as an undergraduate student, Xu says moving from academia to industry felt like a bigger change than moving countries. She compares the broad scope of academic work to the more focused work she’s been doing at Merck. In academia, “we start from a molecular design and go all the way to preclinical studies”, she says, whereas at Merck, she appreciates being able to focus on just one part of the scientific process. — Felicity Nelson
JIE TANG: Vaccine nanoparticles
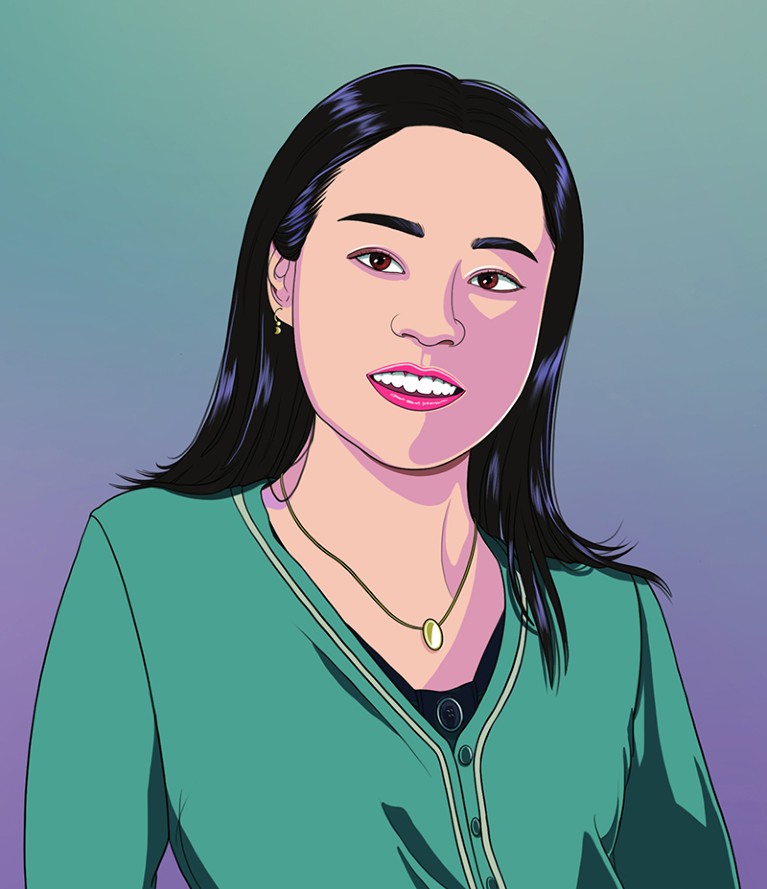
Illustration: Janelle Barone
With her team at Monash University in Australia, Jie Tang is developing an affordable vaccine for colorectal cancer that can be swallowed as a pill. It’s no small feat, she says, because the digestive tract rapidly degrades and clears anything that is swallowed, which doesn’t leave much time for an oral vaccine to help train the immune system. To overcome this challenge, Tang added a special ingredient to the pill: a spiky silica-based nanoparticle4 that sticks to the lining of the gut, ensuring that it stays in place for about 12 hours — long enough to deliver the vaccine’s antigens. Tang was inspired by pollen grains and bristly seeds that stick to clothing and fur, and which are similar to the hair-like surfaces on viruses that help them attach to cells. She first developed these nanoparticles in 2021 as a postdoctoral student at the University of Queensland in Australia, before refining the design at Monash.
The spiky nanoparticles help the vaccine’s antigens to penetrate immune cells and ‘teach’ T cells to recognize and attack cancer cells that have similar antigens. At the same time, the nanoparticles disrupt the ion balance of immune cells called dendritic cells, causing some of them to die off. This carnage prompts other immune cells, such as T cells and natural killer cells, to rush to the site, further boosting the body’s immune response.
Silica nanoparticles have a wide range of uses, from drug delivery to biomedical imaging and electronics. The ones used for drug delivery mainly act as inert vehicles for medications, says Tang. “Our spiky silica nanoparticles are engineered for immune activation,” she says. “They are modified with surface chemistry that enhances vaccine response.” Tang adds that the nanoparticles remain stable within the gut fluid and can penetrate the protective layers of the intestinal tract to reach the underlying immune cells. Silica nanoparticles are cheap and easy to make, she says, so make a “good candidate for scalable vaccine technology”.
These nanoparticles could also prove valuable in agriculture, says Tang. They can be stuffed full of antimicrobials, such as the protein lysozyme, and fed to livestock — a feature that US animal nutrition company Ridley is currently exploring in collaboration with the University of Queensland.
Before her work at Monash, Tang was involved in creating a different spiky silica-based nanoparticle — this one characterized by its hollow interior5. Developed as part of a project led by materials scientist Chengzhong Yu at the University of Queensland, the nanoparticle’s cavity can be filled with large biomolecules, such as DNA and RNA, that have been engineered to influence cellular behaviour and gene expression at targeted locations.
The nanoparticle has been patented by the university and has attracted strong interest from industry because it can be customized, says Tang. In 2018, N4 Pharma and AstraZeneca used the technology to develop Nuvec, a vaccine-delivery system for treating cancer and other diseases, which is in preclinical testing.