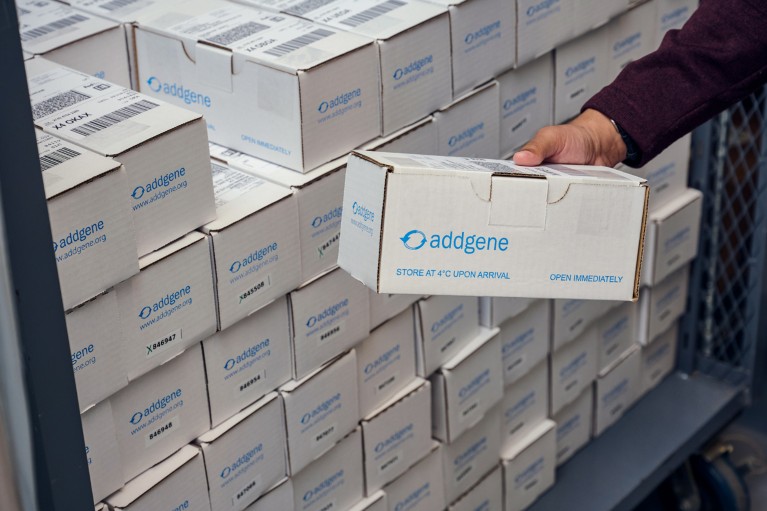
DNA-based materials ready for shipment from the repository Addgene, a service that helps scientists to share plasmid tools.Credit: Matt McKee for Addgene
As a doctoral student in the early 2000s, Melina Fan was at an impasse. She was investigating proteins involved in diabetes and obesity and needed to verify that around 20 proteins she’d identified in the literature would behave reproducibly in her experiments. Researchers coax cells into making proteins they don’t normally express using DNA tools called plasmids. She wrote to each study author asking them to send a sample of their published plasmid for her own research. Barely half replied to her inquiry, Fan recalls, and those who did took months to send the requested materials.
It’s a story that’s regrettably familiar across the life sciences, in which plasmids are ubiquitous tools. Plasmids are circular pieces of DNA that can be manipulated to include the coding sequence for essentially any gene, from fluorescent proteins and gene-regulatory molecules to artificial enzymes. Researchers can then introduce these plasmids into cells to express the encoded proteins.
It can be time-consuming and expensive to build a new plasmid from scratch — hence the practice of asking a colleague whether they can spare an aliquot or two. But, as Fan found, these laboratory-made reagents can arrive late or be unfit for purpose – and the sharing labs must still sort out the legal transfer agreements.
Trove of CRISPR-like gene-cutting enzymes found in microbes
“I realized that there is a big problem with sharing that directly impacted where I was able to take my own research,” Fan says. So, in 2004, she and her colleagues launched Addgene, a non-profit repository based in Watertown, Massachusetts, to make it easier for scientists to access DNA-based materials by taking care of the logistics. Those who develop a popular plasmid tool can offload the hassle of handling requests to Addgene for free. Meanwhile, scientists who order these reagents can count on receiving materials on time and up to standard.
On receiving a new deposit, staff members at Addgene first analyse the sample for quality control and handle all the materials transfer contracts. Fan, who is now Addgene’s chief scientific officer, says that about 30% of the plasmids it receives contain portions that don’t match the expected sequence, so her team works with each lab to resolve any discrepancies. Next, the repository stores the plasmid in its archives and adds it to the online catalogue, which sets prices to help cover Addgene’s operational costs (most plasmids cost academic users $85 each).
Twenty years post-launch, Addgene maintains a library of more than 147,000 plasmids and has shipped more than 2 million samples to 111 countries. Fan and her colleagues provided a list of the ten most frequently requested plasmids, as well as rising stars from the past five years. Here, Nature delves into these tools to learn what makes them stand out from the rest.
Deft delivery
The first step to expressing a gene of interest is getting it inside the cell. One common strategy is to package the desired sequence in an artificial virus that can inject its payload directly into the target cells; several of Addgene’s greatest hits are particularly effective at doing just that.
These vectors are based on a family of viruses called retroviruses that not only inject plasmids into target cells, but also instruct cells to incorporate the plasmid genes into their genomes. But before the mid-1990s, retrovirus vectors could only infect dividing cells, which limited their applications, says Didier Trono, a molecular biologist at the Swiss Federal Institute of Technology in Lausanne, Switzerland. That’s when Trono and his colleagues turned to a type of retrovirus, called a lentivirus, that’s known for its more-expansive infective abilities.
Collection: genome editing
Trono and his team engineered a system based on the lentivirus HIV that can infect cells across a broader spectrum of their life cycles. They used three separate plasmids, two of which encode portions of the virus whereas the third contains the gene of interest. Using this system, the researchers installed lasting mutations in the genomes of human-derived cell lines and in the neurons of living rats1, which had not previously been possible.
Trono knew immediately that this “very cool” system would have widespread applications, which today include cancer immunotherapies, stem-cell research and genome editing, among others. Indeed, the repository’s two most frequently requested plasmids —called pMD2.G and psPAX2 — are from Trono’s lab and are almost universally used for lentiviral expression.
Trono remembers a time before Addgene when requesting plasmids “was a nightmare” — it was a full-time job for a technician in his lab to fulfil all the sample requests they received. Addgene has now sent out more than 40,000 orders on his behalf, or around 250 per month. The repository, he says, “has made a tremendous difference for the community”.
The rise of CRISPR–Cas9
CRISPR–Cas9 is a naturally occurring mechanism that helps bacteria to fight off invading viruses. Researchers Emmanuelle Charpentier at the Max Planck Unit for the Science of Pathogens in Berlin and Jennifer Doudna at the University of California, Berkeley, were awarded the 2020 Nobel Prize in Chemistry for transforming this discovery into a revolutionary, programmable gene-editing tool2.
Their optimized CRISPR–Cas9 editing system is driven by two components: a DNA-snipping enzyme called Cas9, and a short segment of RNA that homes in on a specific sequence to cut. When this ‘guide RNA’ binds to its target, the Cas9 protein makes a precise, double-stranded cut in the DNA sequence. The host cell then tries to repair the damage, but often imperfectly — including introducing mutations that disable the gene entirely. That makes CRISPR–Cas9 particularly suitable for studying what happens when genes turn off. Charpentier, Doudna and their colleagues published their results2 in 2012, and plasmids encoding the engineered components were quickly made available through Addgene.
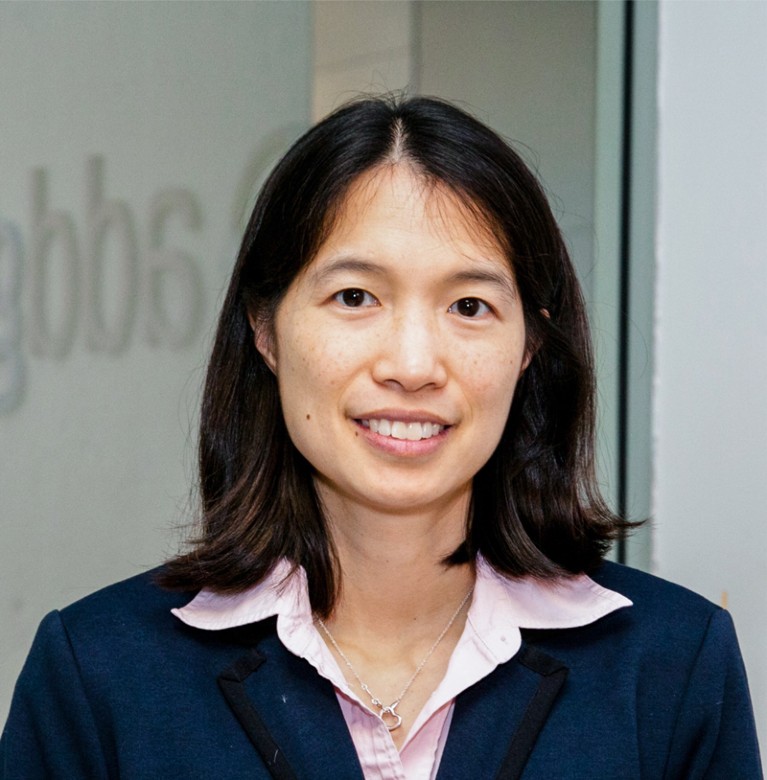
Melina Fan and her colleagues founded the non-profit plasmid repository Addgene in 2004.Credit: Addgene
And that spirit of sharing has continued alongside CRISPR development. Molecular biologist Feng Zhang at the Broad Institute of MIT and Harvard in Cambridge, Massachusetts, and his colleagues, for instance, created tools to make CRISPR–Cas9 editing easier in mammalian cells. Indeed, five of Addgene’s ten most distributed plasmids come from Zhang’s lab, including a Cas9-specific lentiviral vector called lentiCRISPR v23.
Fan says that scientists often submit their reagents to Addgene well before a paper publishes, so that researchers can order plasmids the day the findings go public. The rapid availability of CRISPR plasmids from a centralized distribution hub meant that any researcher — regardless of professional connections or geography — could play with these innovative tools in their own lab, says Samantha Zyontz, an economist and intellectual-property expert at Boston University in Massachusetts who studies the spread of innovative technologies. This availability, in turn, led to faster adoption and adaptation of the technology than might have occurred without the open repository4. “You cannot tell the story of CRISPR without Addgene,” she says.
CRISPR remixed
The CRISPR universe now encompasses myriad Cas variants that can insert, delete, mutate, modulate and even rewrite gene sequences — “like a community toolbox of all these scientists working together”, Fan says.
And several of the hottest commodities from that toolbox were contributed by David Liu, a chemical biologist at the Broad Institute, and have been requested more than 26,000 times, Liu says. “Addgene plays a critical role in maximizing our ability to share constructs developed in our lab with researchers around the world,” he says. His lab’s prime-editing system is particularly popular.
Serious errors plague DNA tool that’s a workhorse of biology
Like canonical CRISPR–Cas9 editing, prime editing uses RNA to guide Cas9 to a specific site in the genome. But Liu’s prime-editor complex nicks only a single strand of the DNA double helix; a second enzyme then replaces the old sequence with a new one, allowing for more-subtle genetic changes5. “Prime editing can mediate all possible base-to-base conversions, small insertions and small deletions in a variety of cell types and animals, making it a broadly useful research tool,” Liu says.
The technique could also spur new treatments for genetic diseases such as cystic fibrosis, says Marianne Carlon, a molecular biologist at the Catholic University of Leuven (KU Leuven) in Belgium. This disease can arise from multiple mutations in the gene for an ion-channel protein that is crucial to clearing airways of mucus and inhaled debris.
Carlon and her colleague Mattijs Bulcaen led a team that combined prime-editing plasmids from Liu with Zhang’s CRISPR-specific lentiviral vector and their own plasmid tools in human cell models of cystic fibrosis. With these plasmids, they were able to rewrite two disease-causing mutations that previous gene-editing methods had failed to correct6. They have now deposited the plasmids from their study with Addgene, and these have already been requested through the repository.
Characterizing the coronavirus
As the COVID-19 pandemic raged, many researchers were eager to turn their expertise towards investigating the coronavirus SARS-CoV-2. Addgene became a go-to resource for plasmids containing nearly every protein in the virus’s genome, including one popular plasmid, called pcDNA3.1-SARS2-Spike, that encodes the protein required for viral entry into cells.
Fang Li, a researcher at the University of Minnesota in Saint Paul, and his team created this plasmid in early 2020 to study the spike protein in isolation, without the other viral components that cause disease. This made it possible for them to identify key structural features in the viral protein that helped to explain why SARS-CoV-2 binds more tightly to human cells than do other known coronaviruses7,8. So far, the plasmid has been cited in more than 50 studies in which other researchers used the isolated spike protein to model the infectious mechanisms of the Omicron subvariants and to understand how COVID-19 affects different tissues.
Today, Addgene’s COVID-19 collection includes more than 3,000 plasmids — around 1,800 of which were designed by Boston-based Ginkgo Bioworks, a biotechnology company that provides research and development services. Brad Chapman, a principal data scientist at Ginkgo, says he and his colleagues realized early on that they had the resources to design and generate plasmids that could express key viral proteins for a broad variety of experimental conditions. However, he notes, the challenge of obtaining reagents from other researchers is often so onerous that it impedes reuse and building off existing work. Despite the sizeable load of new tools from Ginkgo, he says, Addgene seamlessly made the materials open and accessible.
Painting plants red
Among the most colourful constituents in Addgene’s recent hits is 35S:RUBY, a plasmid that turns verdant plants a vivacious red. Yunde Zhao, a plant biologist at the University of California, San Diego, and his colleagues crafted the plasmid to help identify plants that had successfully undergone CRISPR gene editing. Inspired by the rainbow of colours on display in a supermarket’s fresh-produce aisle, Zhao and his team set out to create a vibrant marker that would be visible at a glance without damaging the plant. They turned to betalain, a molecule that gives beetroot, dragon fruit and Swiss chard their vibrant hues.
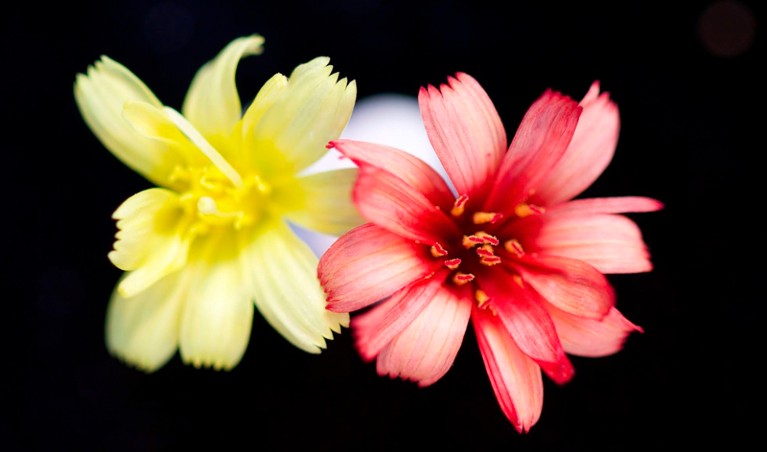
The RUBY system turns plants red to help identify those that have undergone CRISPR editing.Credit: Beth Rowan, Michelmore Lab, UC Davis Genome Center
In nature, betalain biosynthesis is a multistep process that requires several genes. So, Zhao and his colleagues developed a synthetic sequence called RUBY that streamlines the enzymatic components into a single artificial gene. When the researchers introduced this into rice plants, the crops blushed red within days9. The feature could help researchers to monitor genetically modified crop fields, because the colour is noticeable from a distance, Zhao says. He and his collaborators even invented a red turf grass that could be used to grow team logos directly on sports fields.
Zhao says that Addgene has fulfilled more than 700 orders of his lab’s plasmids since they were deposited in 2020. The results can be seen in an online photo gallery that Zhao curates of ‘RUBY-fied’ plants shared by researchers from around the world (see go.nature.com/3mvrpkf).
Biologists Midori Tabara and Kazuki Motomura at Ritsumeikan University in Kusatsu, Japan, used 35S:RUBY to update a fundamental technique in plant biology for measuring RNA silencing10. Many biotech firms are interested in engineering plants to synthesize complex molecules. But because plant immune systems try to degrade foreign genetic material, scientists must monitor RNA-silencing activity in their experiments — often using fluorescent proteins, says Tabara. RUBY makes those measurements faster and easier by eliminating the need for specialized equipment. “RUBY is a great tool,” Tabara says, especially for working with students. “It is very strong visually and very affordable.”
Improving cancer immunotherapy
Addgene reagents are also making inroads in oncology research.
Humans have specialized immune cells called T cells that help to fight off infections. The cells are coated in receptor proteins that bind to molecules, or antigens, on the surface of infected cells. When these receptors find their target antigen, the T cell destroys the offending cell.
Researchers can equip a person’s T cells to home in on the subtle surface markers present only on cancer cells, using ‘chimeric antigen receptors’ (CAR). However, CAR molecules are not one-size-fits-all, and researchers are still unpicking the basic science that governs which configurations are most effective. The modular design of a plasmid called pSLCAR-CD19-BBz — deposited by Scott McComb, a research officer at the National Research Council Canada in Ottawa, and his team — makes it easy to swap out the antibody components to find the best cancer-killing candidates11.
NatureTech hub
Denis Wirtz, a biomolecular engineer at Johns Hopkins University in Baltimore, Maryland, and his team, for instance, had previously developed synthetic proteins called velocity receptors that make cells more mobile. By modifying McComb’s plasmid to express a CAR that recognizes an antigen found on many types of solid tumour, Wirtz and his team showed that their velocity receptors improved the infiltration abilities of CAR T cells12. “Starting from scratch would have been very, very difficult,” says Wirtz. “We would have been stuck.” The findings were posted on the bioRxiv preprint server in March and are undergoing peer review.
And that’s just one research team. McComb says his plasmids have been distributed to more than 450 labs in 38 countries, which is one way in which he quantifies the impact of his research on his CV and in funding proposals. “It’s an easy way for scientists to show their value to the community,” he says.