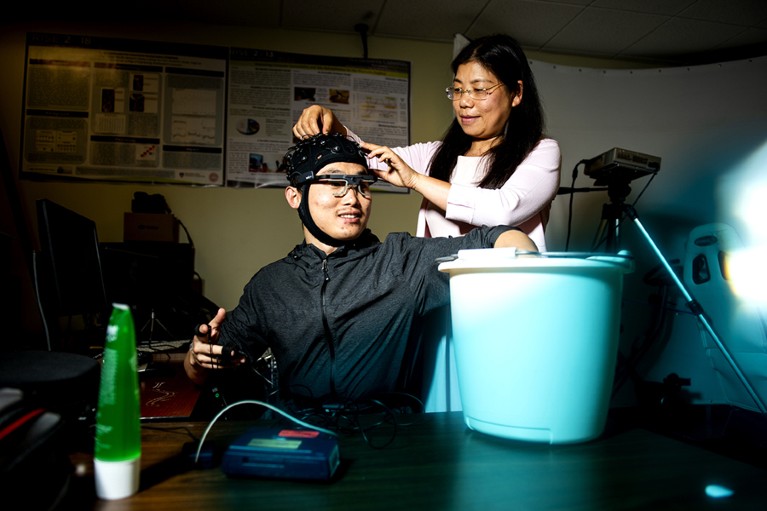
A participant holds his hand in a bucket of ice while Yingzi Lin monitors his pain response.Credit: Ruby Wallau/Northeastern Univ.
The graduate student bears down on my arm with a force akin to a firm handshake. This pressure might not seem like much, but when concentrated on a patch of skin roughly the size of a small coin, the sensation gradually starts to hurt.
As discomfort escalates to pain, a sensor strapped to my chest detects changes in my heart rate, breathing pattern, skin conductance and other bodily responses. These physiological signals are processed through advanced algorithms to generate a pain score. Displayed on a smartphone app, my pain level is 4.
Had you asked me to rate my pain on a scale of one to ten, I would have had no idea how to convey my impressions accurately. Yet, this kind of deeply personal, highly variable and imprecise self-reporting is exactly what most clinicians rely on.
Nature Outlook: Pain
Such scores are influenced by a multitude of factors, including a person’s medical history, gender, culture and emotional state; these can all affect how an individual responds to pain, and can mask — or amplify — the physiological response. This can lead to misdiagnosis or inadequate treatment, because the intensity or nature of the pain experienced is not reflected accurately.
Moreover, many individuals — including babies, people who are non-verbal, people who are critically injured and those under sedation — cannot communicate their symptoms effectively. This further complicates the management and treatment of pain, and hinders the delivery of appropriate analgesia.
To overcome these challenges, many researchers now aim to bring a level of objectivity to the diagnosis of pain, through devices designed to eliminate the social and individual variables that contribute to the current inaccuracies. “People have been interested — desperate, really — for an objective measure of pain in humans for a long time,” says Jeffrey Mogil, a pain researcher at McGill University in Montreal, Canada. “It’s a huge priority.”
If a reliable biological readout existed, clinical trials that evaluate new drugs or devices could continuously monitor the pain of participants, eliminating the subjective noise and biases that are inherent in survey results. And in routine medical practice, improved diagnostics could lead to treatment plans that are free of prejudice or disbelief about whether someone might be faking or underselling their symptoms. So far, no method for quantifying pain is definitive, and the diagnostic technologies under consideration vary. Some rely on brain imaging. Others involve measurements of bodily signals, such as pupil dilation, facial expressions or levels of certain biomarkers in the blood.
At the laboratory I visited at Northeastern University in Boston, Massachusetts, mechanical engineer Yingzi Lin and her colleagues at are collecting a wide range of physiological parameters. As well as information of the kind presented by the sensor that I wore, they are gathering eye-tracking and electrical-brain-activity measurements to identify a reliable signature of pain. The task is to extract meaningful patterns from this jumble of biological outputs, each of which is indicative, in its own way, of the pain experience. “It’s a lot of data,” Lin says. “It’s a challenging problem.”
Compounding the difficulty, pain is rooted in individual perception. Consequently, the most accurate understanding of pain can come from only those experiencing it, provided they can articulate their feelings.
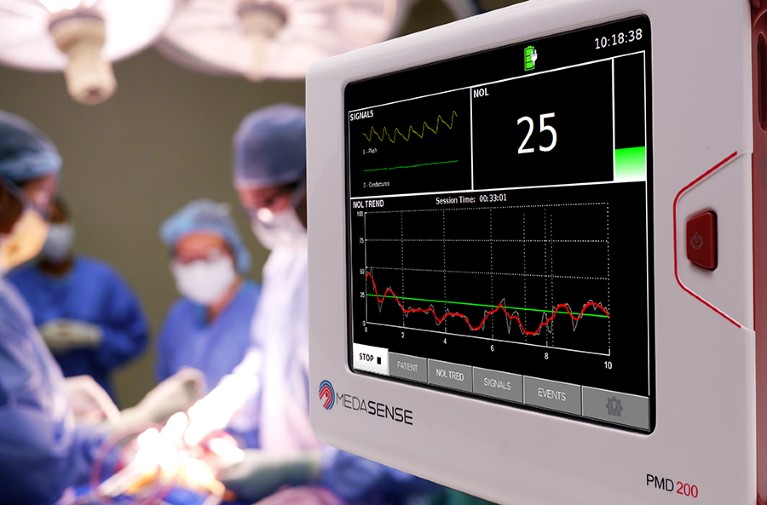
The PMD-200 bedside monitor is the only device authorized by the US Food and Drug Administration for the measurement of pain.Credit: Medasense
The field is therefore caught in something of a catch-22: striving for objectivity in describing an intrinsically subjective experience. That doesn’t make the pursuit of pain biomarkers futile, however. As Carl Saab, a neuroscientist at the Cleveland Clinic Lerner Research Institute in Ohio, points out, a reliable measure could still help clinicians to better assess, classify and track pain, while also considering self-narratives about the intensity and severity from the person experiencing pain.
“We cannot disregard the patient’s report. We have to augment it,” he says. “It’s not either/or. It’s going to be both.”
Last year saw the introduction of the first device in the US health-care market designed to provide a quantitative assessment of pain based only on the body’s physiological responses to pain signals — referred to as nociception. The device, from Medasense Biometrics in Ramat Gan, Israel, features a finger probe that gathers information on a person’s heart rate, skin moisture, movement and temperature, and produces a score from 0 to 100.
The platform is authorized in the United States only for use during surgery with people who are under anaesthesia and unable to communicate their pain levels. By allowing clinicians to adjust painkiller dosages in line with an individual’s pain, it aims to optimize pain management and mitigate the risks associated with unnecessary opioid prescriptions.
Probing pain
During surgery, the technology can reduce the administration of opioid painkillers; it can also lower pain scores when individuals are in recovery from major operations. Now researchers are exploring whether the finger probe can aid with pain monitoring in other patients — in the intensive-care unit, for example, or among people with complex regional pain syndrome. “It’s not limited to the operating room,” says Medasense founder and chief executive Galit Zuckerman Stark, noting that her team is now refining the tool’s algorithms to more accurately capture the types of pain experienced by fully conscious individuals.
Others, meanwhile, are turning to brain-imaging technologies. Using functional magnetic resonance imaging, for example, several groups have identified patterns of brain activity that reflect different types of pain — including sensory, emotional and cognitive aspects of the experience. And some researchers have achieved similar results with electrophysiological recordings.
These efforts have yielded some of the most accurate pain-related signatures so far, offering insights into the neural pathways associated with pain and highlighting targets for therapeutic interventions. “This lays the groundwork for identifying potential treatment targets,” says Tor Wager, a neuroscientist at Dartmouth College in Hanover, New Hampshire.
But the cost and complexity of brain-recording technologies make these methods unsuitable for clinical use. “It has to be practical and portable,” Saab says — which is where simpler diagnostic tools and wearable devices come in.
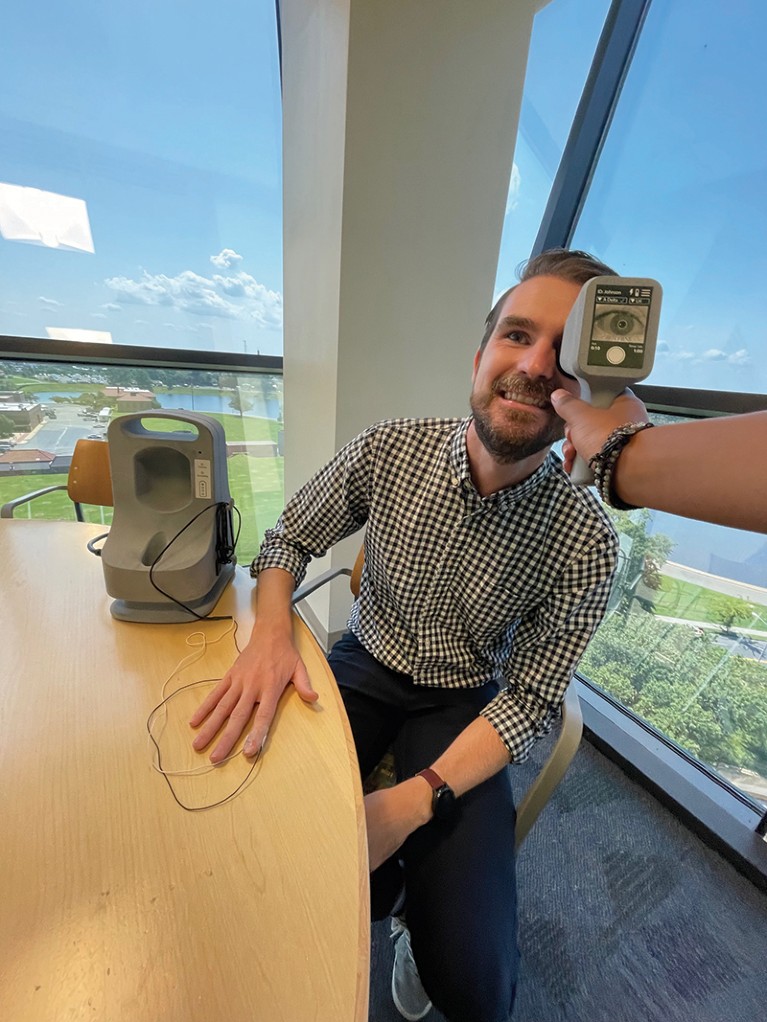
The AlgometRx device can create a pain profile from scanning someone’s eyes.Credit: AlgometRx, Inc.
Julia Finkel is a paediatric anaesthesiologist at the Children’s National Hospital in Washington DC who studies pupil responses to pain. In her lab, and through a company she founded called AlgometRx, also in Washington DC, Finkel has developed a handheld device that scans someone’s eye and measures pupil dilation in response to gentle electrical stimuli applied to a finger or toe. The system takes advantage of three electrical frequencies, each of which activates a different type of sensory nerve fibre that relays signals to the brain, causing the pupils to widen. According to Finkel, the precise nature of this reflex depends on the intensity and type of pain that an individual is experiencing at the time of stimulation — and her device can distinguish those differences and create a pain profile accordingly. Finkel and her colleagues have validated the platform in people with inflammatory pain from conditions such as the autoimmune disease lupus, as well as neuropathic pain that stems from treatment with chemotherapy drugs.
Keeping tabs
AlgometRx’s technology provides a snapshot of a person’s pain at a point in time. That’s fine for some situations, such as an assessment by a physician, but it can’t track fluctuations in pain levels over extended periods.
In an effort to provide continuous pain monitoring, CereVu Medical, a start-up firm in San Francisco, California, has developed a small, wearable patch that sticks to the forehead and measures neuronal activity in real time by tracking variations in blood flow at the surface of the brain. Proprietary algorithms translate these changes into an objective pain index that CereVu scientists have shown correlates with subjective pain scores reported by people receiving epidural steroid injection for the treatment of chronic pain (M. Orzabal et al. Int. J. Environ. Res. Public Health 19, 17041; 2022). A larger trial involving 130 women experiencing labour pain during childbirth also indicates that the device performed well, matching women’s description of their own pain around 80% of the time, according to unpublished data.
The US National Institutes of Health has also joined the hunt for pain metrics and wearable technologies. Through an initiative called Helping to End Addiction Long-Term (HEAL), the agency has spent nearly US$50 million on projects aimed at discovering and validating biomarkers connected to various pain conditions, including musculoskeletal pain, pain associated with the inherited disorder sickle cell disease, post-traumatic headache, pancreatitis, eye pain and chemotherapy-induced pain.
“Right now, there are no validated biomarkers for pain,” says Ramachandran Arudchandran, who leads the HEAL biomarker effort at the National Institute of Neurological Disorders and Stroke in Bethesda, Maryland. “However, recent research has identified some promising biomarkers that are providing new insights into how pain works” — and several candidate metrics identified through HEAL are advancing through analytical and clinical validation.
Lin has been fine-tuning her platform called Continuous Objective Multimodal Pain Assessment Sensing System (COMPASS) using data from people with chronic low back pain to validate her group’s machine-learning algorithms. Such artificial-intelligence tools have proved adept at synthesizing diverse data sets to extract valuable insights that align closely with people’s own pain assessments, surpassing the capabilities of any single modality.
More from Nature Outlooks
In a study of cold-induced pain, for example, the researchers found that an algorithmic inference gleaned from nine measures outperformed other approaches — including a more limited subset of biometric indicators and a model derived from only facial expressions and brain-activity signals — when evaluated against self-reported pain scores (Y. Lin et al. Front. Neurosci. 16, 831627; 2022).
Outside the lab, however, running such a battery of physiological tests is impractical. Lin has, therefore, been working to streamline the system and concentrating on the key modalities that most accurately reflect pain levels. Her aim is to identify three or four crucial indicators. This refinement is necessary, she says, to ensure the tool’s practicality in health-care settings.
When I tried the cold-exposure task in her lab, I had only one sensor on my body feeding data into COMPASS. Facial tracking, measurements of pupil diameter and monitoring of brain activity weren’t recorded.
The platform proved glitchy. With my hand submerged in a bucket of ice water, the objective pain scores displayed on the app generally trended upwards. The readings were inconsistent, however, fluctuating as I experienced a steadily increasing sensation of pain.
“Hmm, it’s not really working,” says Lin, acknowledging that much optimization work remains. “I think maybe we should stop.” As I withdraw my hand from the icy cold, the pain quickly subsides — and I don’t need an objective measure to tell me when, after a few moments, the pain is gone.